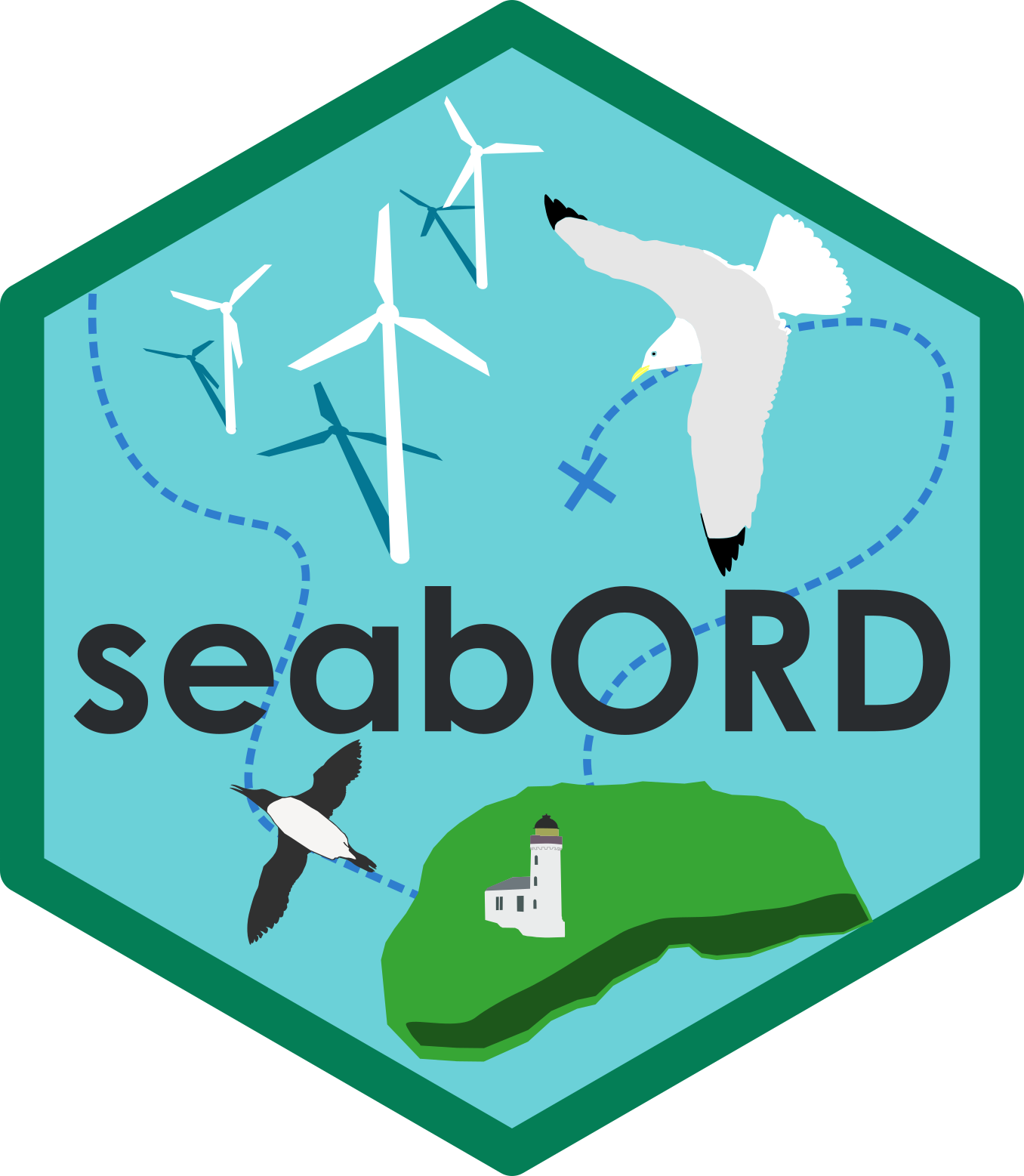
Overview of seabORD
B_explanation_model.Rmd
library(seabORD)
Model purpose
The purpose of the model is to predict the demographic impacts on seabirds of sub-lethal displacement and barrier effects resulting from interactions with ORDs, evaluated through predicted changes to adult mass, adult survival and productivity. Demographic impacts are derived by estimating alterations to time/energy budgets of four different seabird species during the chick-rearing period, when exposed to ORDs within their foraging ranges. To estimate the impacts of ORDs, baseline scenarios (no ORD footprints included) are compared with scenarios containing one or more ORDs, providing estimates of potential changes to adult mass, adult survival, and breeding success under alternative scenarios of ORD exposure.
In practice
Figure 1 below provides a view of how the model is applied in practice and how it can be used to assess ORD impacts resulting from one ORD, or several in combination (cumulative effects).
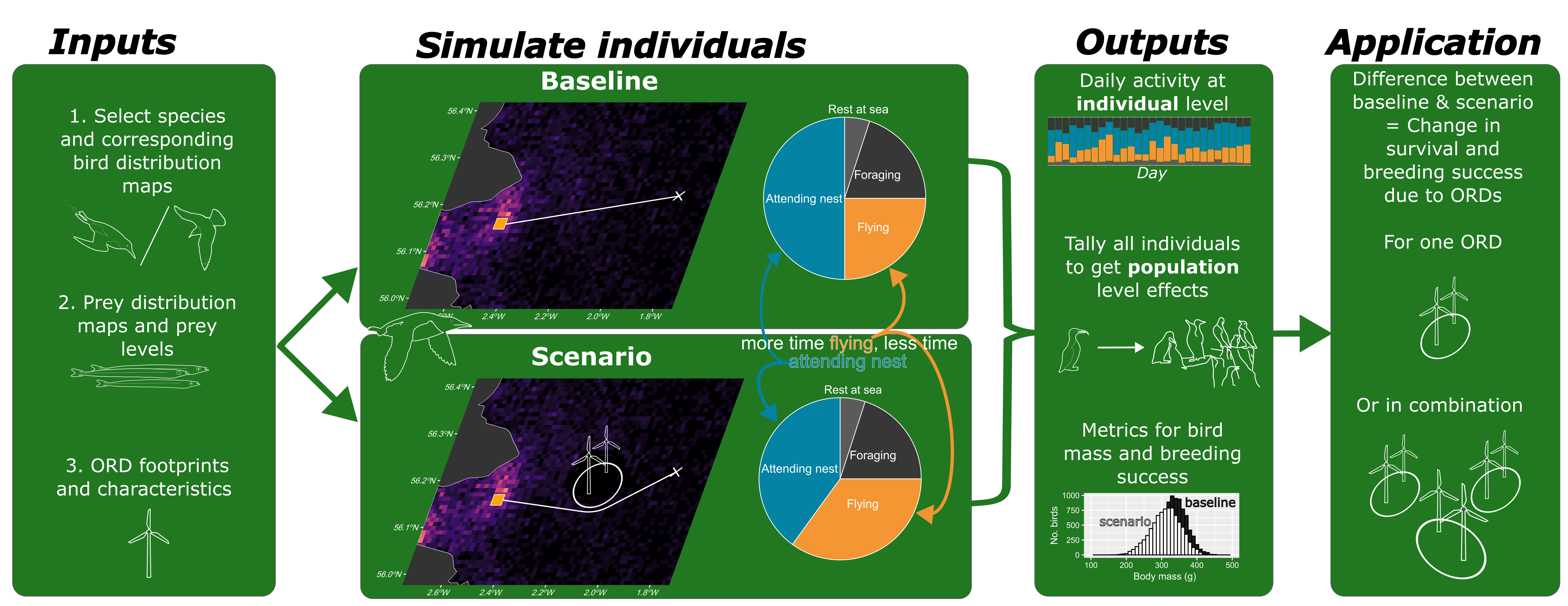
Code structure & processes
The model was developed in R (R Core Team, 2024), and code is provided in GitHub (https://github.com/NERC-CEH/seabORD_pkg).
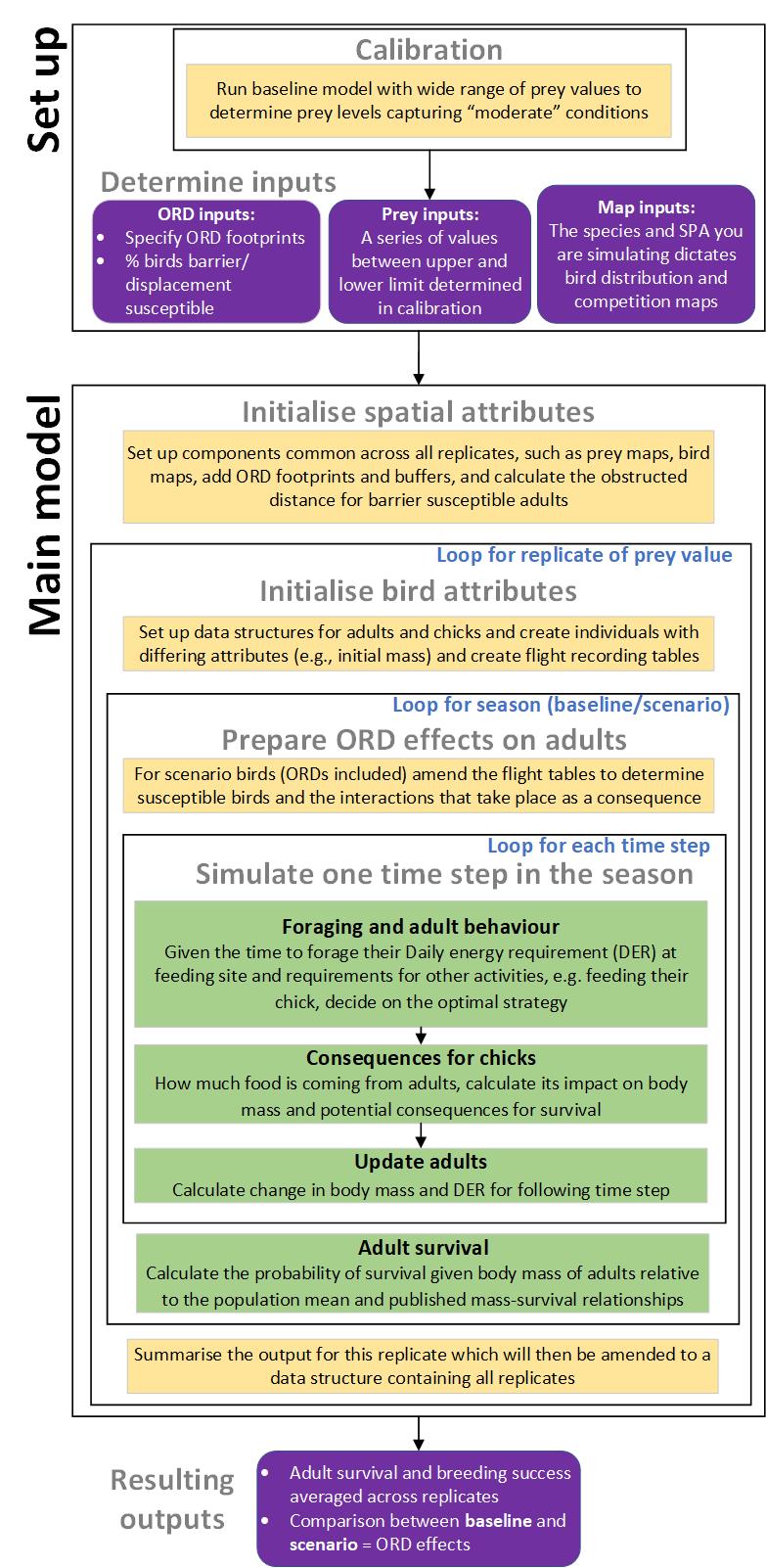
The model can be separated into the set-up process and main model, which has a nested structure (Figure 2). Within each time step the adults make foraging decisions and then based on the outcomes of those decisions, a pair’s chick’s condition is updated depending on whether its parents managed to catch enough prey to feed themselves and their chick, with prioritisation of themselves occurring when constraints imposed by foraging conditions and/or experienced body condition are sufficiently bad. Names in bold refer to the submodels within these processes.
Foraging and adult behaviour – At the beginning of a time step, each adult is assigned a location for feeding during each foraging trip from the colony based on bird density maps. The prey available in this foraging location is used to calculate the time taken to forage their required amount in this time step (calc_foragecapture) using an intake rate based on a Type II functional response (Holling, 1959), with an adjustment to account for the effect of competition from conspecifics on individual instantaneous intake rate. Using this information in conjunction with knowledge of associated activities such as flying to and from the foraging site (including barrier effects of flying around the ORD), plus a knowledge of their own condition and whether or not their chick is alive, each adult then chooses the optimal foraging strategy for this time step (calc_strategy), which is based upon attempting to acquire their required energy intake for the day, whilst minimising time spent away from their chick to avoid unnattendance. This determines how many trips the adult will undertake in this time step and what behaviour the adults exhibit with respect to their chick, such as potentially leaving them unattended or abandoning the breeding attempt due to constraints.
Consequences for chicks – The amount of food captured by each adult during the time step is then converted to energy shared between themselves and the chick and summed to estimate the energy provided to the chick. The resulting increase in chick body mass, based upon food provided by both its parent, is calculated and compared to a corresponding optimum body mass for a chick at the given stage of the season (calc_chickcare). If this value falls below a defined threshold the chick starves and suffers mortality. At this point the consequences of the adults’ behaviour towards the chick determine whether the chick remains alive, or if it dies through abandonment, a parent dying, which is followed by several stochastic processes relating to other potential causes of death: unattendance by the parents (calc_unattendance), other mortality from causes like storms (calc_othermortality), or in the case of puffins, mortality from predation via hunger driving them to the entrance of the burrow (calc_puffinmortality).
Update adults – Each adult’s state variables are then updated with the chick’s status, which can result in a change in the feeding mode if their chick dies, having consequences for their foraging strategy in the next time step. Adult body masses are then updated based on the energy gained and expended foraging and in other activities (calc_adultbmchange). Daily energy requirement for the following the following time step is then calculated (calc_adultdee).
Adult survival – Upon completion of all time steps for each season (baseline/scenario) within each replicate, adult survival over the subsequent winter period is estimated using the mass of each adult which survived to the final time step, representing the end of the chick-rearing season. Survival is estimated using relationships from previously published studies (Oro and Furness 2002; Erikstad et al. 2009), by converting the mass of each adult into a probability of survival via mass-survival relationships (calc_pSurvival). This probability is then passed to a stochastic process which dictates which individuals survive according to their relative probabilities.
ORD interactions
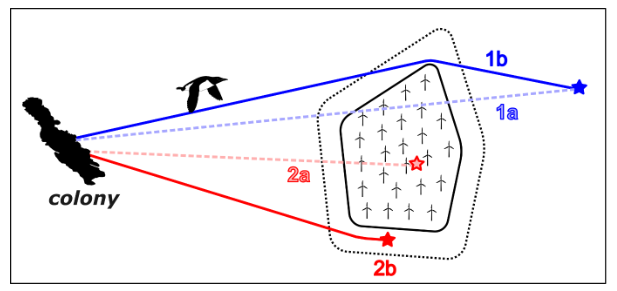
Adult behaviour concerning sublethal interactions with ORDs is split into two widely accepted potential impacts, barrier and displacement effects. A barrier effect is thought to occur when a bird is attempting to access a foraging site from the colony where the most direct path is obstructed, such that avoiding the ORD results in increased travelling distance with potential energetic consequences. Barrier effects operate within the model by adjusting baseline flight paths (when no ORDs are present) using the shortest path directly around the ORD in the scenario run (Figure 3, 1b). A displacement effect occurs when an individual is excluded from foraging in a potentially productive area within an ORD footprint. The model represents this by displacing a bird from its previously chosen foraging location inside an ORD footprint into a new location in the displacement zone, with new foraging locations chosen proportional to the probability of bird usage within the displacement zone (Figure 2, 2b).